“Cumulative Fatigue Failure for Dummies”
I broke a crankshaft while racing my 100S and that experience always haunted me because original 100S engine blocks are extremely rare and I knew that I had been very lucky not to have destroyed mine with that “blow up”.
Over the years I had encountered many cases of 100 crankshaft failures and it was the fear of another in the “S” that caused me to study the issue. The conclusions arrived at and described below prompted me to build a very special competition engine for the car which you can read about here.
WHY DO AUSTIN HEALEY 100 CRANKSHAFTS BREAK?
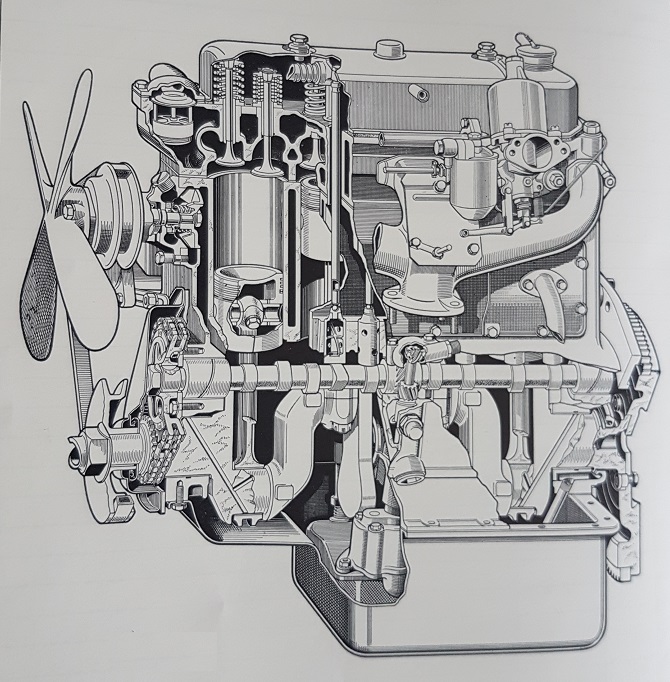
The Austin Healey 100 engine was actually developed from a 1929 Chevrolet design and several aspects of the design contribute to the crankshaft failure problem, these include:
- The engine size which at 2660 c.c., is around the upper limit of what is optimal in an automotive 4 cylinder unit.
- The relatively spindly forged steel crankshaft which has no “pin overlap” and is only supported in 3 main bearings
- The extremely big and heavy flywheel necessitated by the engine design.
To understand why these 3 aspects of the design are major issues it is first important to understand some less than common engineering terms and concepts.
CRANKSHAFT ROTATIONAL SPEED OSCILLATION
Referred to in this article as CRSO simply refers to the changes in the speed of rotation of the crankshaft that occur during each revolution.
The real cause of CRSO is not, as one might initially conclude, the firing pulses of the engine, but more a function of the kinetic energy of the reciprocating parts of the engine, i.e. the pistons and connecting rods.
As the engine runs the mass of each piston and wrist pin and a portion of that of the connecting rod is accelerated to a substantial velocity then decelerated back to stationary twice a revolution. The forces required to do all this work are applied directly to the crankshaft and they result in significant changes in its speed of rotation during each rotation. CRSO is particularly exaggerated in four cylinder engines because those forces, which are proportional to the square of the engine’s RPM, occur at all four crank throws at the same time.
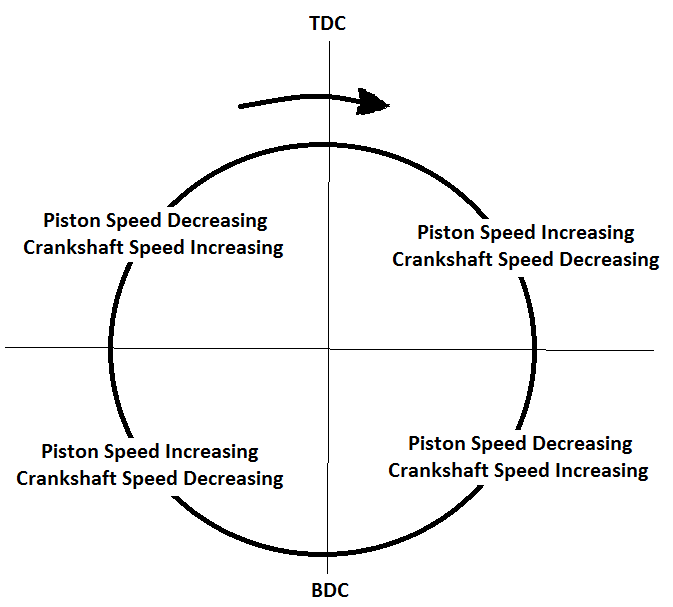
Interestingly the largest force from the “power stroke” of each cylinder occurs exactly whilst the crankshaft is attempting to accelerate the reciprocating parts so actually serves to decrease CRSO but that combustion force is pretty small when compared to the peak torque of around 2400 lb ft that CRSO produces.
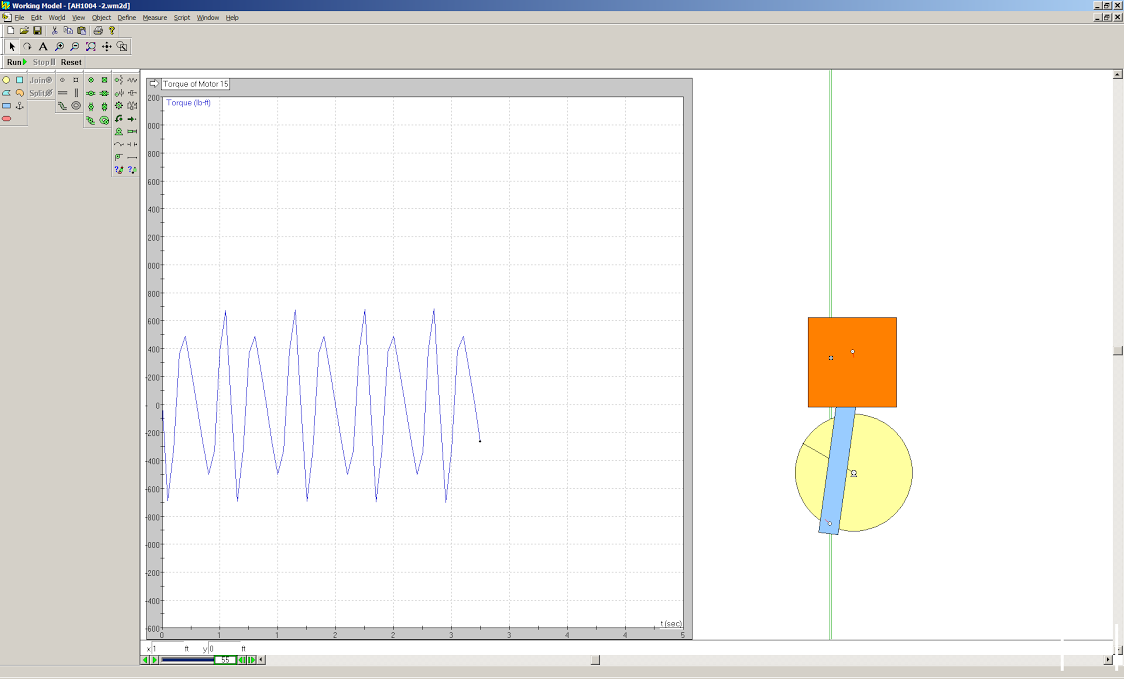
TORSIONAL VIBRATION
One way to think of torsional vibration is to consider a long spring steel rod supported in bearings with one end rigidly fixed and a weight on the other. Turning the weight a little, i.e. twisting the rod, and releasing it will cause the weight to oscillate back and forth under the influence of the spring action of the rod much like the escapement in a clock.
This oscillation will have a set “frequency” regardless of its amplitude (size), or for that matter the speed of rotation of the assembly, and is termed the critical frequency of the assembly.
A very small force applied regularly and in phase with those oscillations can rapidly increase the amplitude of the oscillation much like a series of small pushes on a child’s swing, keep doing it long enough and junior will either fly off into space or wrap round the top bar!!.
In the case of a crankshaft the flywheel acts somewhat like the fixed end of the rod and the CRSO somewhat like the small applied force.
Testing by The University of Southampton’s Institute of Sound and Vibration Research has shown that the 100 crankshaft is very susceptible to torsional vibration particularly over 4500 R.P.M which is not at all surprising considering its “spindly” design.
FATIGUE FAILURE
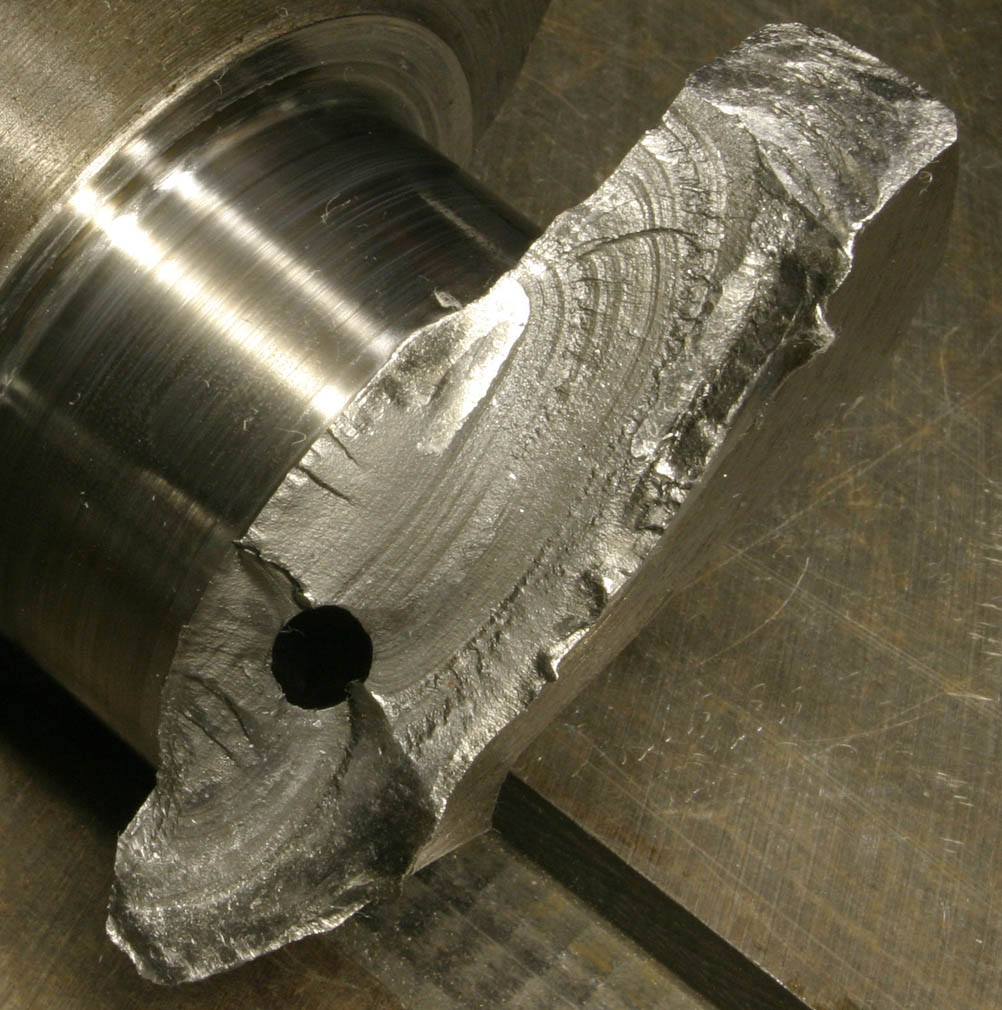
Fatigue failure is defined as “The tendency of a material to fracture by means of progressive brittle cracking under repeated alternating or cyclic stresses of an intensity considerably below the normal yield strength of the material and is a function of the magnitude of the fluctuating stress.” You may be able to tell that I didn’t write that mouthful!!
THE FLYWHEEL’S PURPOSE
Engine designers use flywheels to dampen CRSO and make their designs run more smoothly, particularly at lower speeds. By dampening the CRSO the flywheel also serves to protect the transmission. The flywheel cannot totally remove the crankshaft’s speed oscillations and the elimination of most the remaining is achieved by installing torsional coil springs into the clutch disc.

Unfortunately the problem with using a heavy flywheel, like that in the 100 engine, is that the crankshaft must absorb the oscillations by “flexing” or twisting somewhere near the flywheel causing torsional vibration which in turn produces metal fatigue and ultimately fatigue failure of the crankshaft usually somewhere around the #4 throw. (Refer to pic above…..nasty!).
SO HOW DOES THIS ALL PLAY OUT?
As mentioned above the forces that generate the CRSO increase dramatically with R.P.M. and metal fatigue increases as a function of the magnitude and duration of this fluctuating stress.
All crankshafts have a critical frequency but in general terms with “spindly” crankshafts the critical frequency is lower, at a guess, probably somewhere around 160Hz in the case of the 100 engine.
This is all very bad news for those of us who like to drive our sports cars the way that they were intended to be driven.
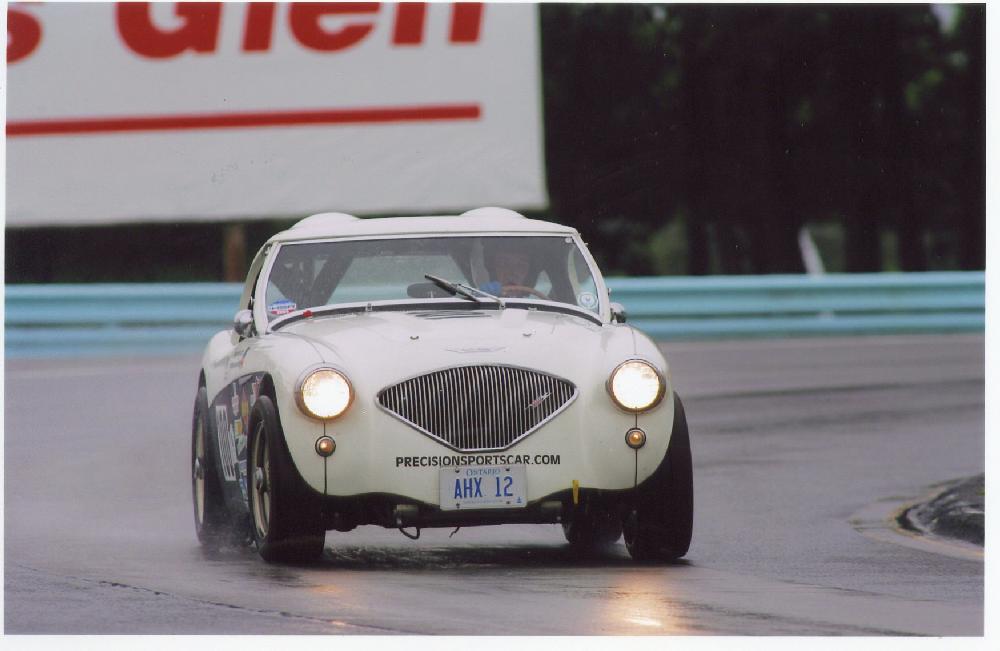
I think the reality is that if you have an Austin Healey 100 with an original equipment crankshaft and flywheel, which you drive it at all spiritedly, sooner or later the crankshaft is going to break somewhere around the rear cylinder. You can take some solace in the fact that usually such a break does not completely destroy the engine but you certainly are not going to be able to gently drive the car home!!!
WHAT IS TO BE DONE?
In no particular order here are some measures that may be considered.
Limit Maximum R.P.M.
Probably the best option, particularly as the 100 engine is a real torque producer and even with an “M” cam will pull strongly from under 1500 R.P.M. however, with a standard diff ratio and 28% overdrive, the engine is usually running between 3000 and 3500 R.P.M at highway speeds which is not good. Changing to a 3.66/1 (spiral bevel) or 3.54/1 (hypoid) differential ratio certainly helps keep engine speeds down as would the very rare 32% overdrive however, eventually the crankshaft will probably exceed its so called “fatigue life” and break.
Replacement Crankshaft.
The first things that most people consider when they have a broken crank is where can I get a stronger replacement and what will it cost?
There are lots of folks out there flogging “steel” or “competition” cranks but there isn’t a lot of information about what these cranks actually are. The debates about the merits of the various manufacturing methods rage on but there is no question that the material used is very important. Top of the line cranks these days are made from EN40B chromium molybdenum or 4340 nickel, chromium, molybdenum or some similar alloy steel so if you could acquire a good quality forged or billet crank made from that it would probably last a long time. Unfortunately just replacing the crank with one of similar dimensions will not do much to minimize the affects CRSO or change the critical frequency because those properties are inherent in the engine and crankshaft design.
Nitriding or “Tuftriding” the Crankshaft.
Nitriding is a process which does not modify the properties of the base material from which the crankshaft is manufactured but it does produce a very hard and durable surface some few thousandths of an inch thick. Whether or not nitriding increases the fatigue life of a crankshaft is however not a “slam-dunk” and is very dependent upon the parent material and the process used. Nitriding however requires that the parent material contains some aluminum, chromium, molybdenum or titanium which apparently a standard 100 crank does not. The original 100S crankshaft was made from EN40B alloy steel which is very strong and ideal for nitriding.
Tuftriding produces a much thinner hardened surface but does not require the specialized parent material.
Lightened Flywheel.
This option will almost certainly decrease the magnitude of the torsional vibration and increase the life of your crankshaft BUT over time the rotational speed oscillations will probably be more than the clutch plate springs can withstand resulting in their failure and, furthermore, the elimination of flywheel inertia will result in the transmission being subject to potentially destructive vibrations. (THINK BN1 GEARBOX FAILURES) Let’s say that “the jury is still out” on that one.
Crankshaft Harmonic Damper
To quote Wikipedia “To minimize torsional vibration, a harmonic balancer is attached to the front part of the crankshaft. The damper is composed of two elements: a mass and an energy dissipating element. The mass resists the acceleration of the vibration and the energy dissipating (rubber/clutch/fluid) element absorbs the vibrations.”
The Austin Healey 100 engine was designed in the days before crankshaft dampers were in common use on 4 cylinder engines. The design of such dampers is a complex process as the damper’s natural frequency (typically 0.8 – 0.9 of that of the crankshaft itself) is critical and therefore unique to the particular application. Installing a damper of the incorrect type can be worse than not having one at all. Before installing one I would want to be very sure that it was correctly designed specifically for the 100 engine. Additionally it should be borne in mind that a damper will do little if anything to minimize CRSO.
A good article on dampers can be found here.
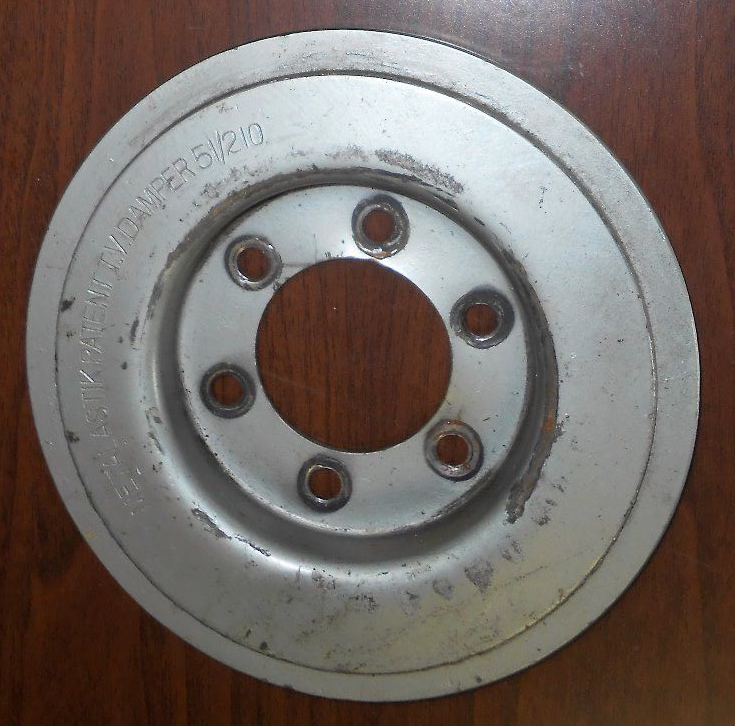
Lightened Rods and Pistons.
Another expensive option which would almost certainly have definite but limited benefits.
Improved Crankshaft Design.
This is only an option for the “serious money” crowd. The much heavier and stronger EN40B nitrided crankshaft from an FX3 Austin Diesel taxi engine can be installed in the 100 block.
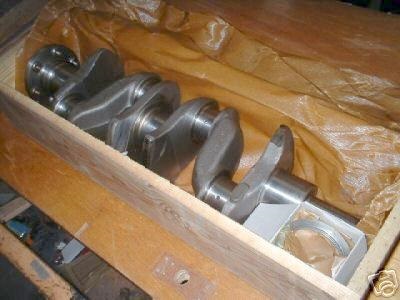
FX3 CRANKSHAFT
To accommodate the much heavier webs the center main bearing is narrower which necessitates some creative machining of the block and the addition of a substantial “strap” to reinforce the narrowed bearing cap. Custom connecting rods and different pistons are required to accommodate the 0.250” increase in the crank pin diameter and 3/8” shorter stroke of this crankshaft. Because of its increased mass a substantially lighter flywheel can be used. This radical modification addresses the inherent design issues with the 100 engine and has proven to produce the basis for a powerful and reliable engine however, the crankshafts are now very hard to find. A custom made billet crank of similar dimensions could be produced.
As they say……”Pick Your Poison”.
Building a race 100 engine any recommends ? George Gabriola isl BC Canada
Hi George, sorry to be slow getting back to you, we are updating our web page and things get lost !!
As my post says the 100 crank is really the weak point of the engine (clamped gudgeon pins would be a close 2nd) and it really is related to the basic engine design and not something that you can “fix” easily.
I would never entertain the idea of building one with a standard crank and even with a billet crank many racers in the UK have suffered failures … there just isn’t enough material there to prevent fatigue.
Taxi cranks show up for sale once in a while, I bought a new one a few years back, and one of those, or a billet version is where I would start.
I experimented the same exact fault in my 1300 Innocenti Mini Cooper engine!
I can send you a picture, the crankshaft broke in the same point (maybe lubrication hole is a weak point?) as the 1.3l engine could be considered a 50% AH100 engine.
I seems as if the BN 1 engine was an obsolete 30’s Chevy design consideration for the early 50’s Austin Healey. Chevrolet was about release in 1955 the small block Chevy V8 engine during the development and release of 100 4 Austin Healey. Even in all iron head and block configuration the Chevy V8 wieghed 100 lbs less than the lumpy BN1 4 cylinder lumper. Purest Austin Healey fans hate the concept of Small block V8 Chevy engine while embracing an obsolete 4 cylinder Chevy Engine design. Go figure. In the mid 60’s had a friend Dave Lyons Edwards AFB. CA. had a 1954 AH with a 327 cid small block Chevy with a munic 4 speed trans. Not only it beat All old & new hot cars it was 4 wheel drifter to boot!